Hitachi Energy – Hitachi Energy invests additional $250 million USD to address global transformer shortage
- More than 40 percent of the total investment dedicated to the U.S. underpins commitment to scale up the industry to meet surging transformer demand
- The U.S. investment includes hiring more than 100 people to expand domestic key component manufacturing to strengthen local supply chain
- Builds on the $6 billion USD investment announced in 2024 – the largest in the industry in recent years to respond to the urgent need to upgrade power grids
Hitachi Energy announced today at CERAWeek additional major investments of more than $250 million USD by 2027 to expand global production of critical components for transformers. This rapid follow-up to the recently announced $6 billion USD investment across the company portfolio reflects the escalating transformer shortage, which continues to increase.
As the electrification of industries, particularly data centers and AI, drives unprecedented demand for electricity, the need for transformers has surged beyond initial projections. To keep pace with this accelerating demand, Hitachi Energy is expanding its commitment to scale up production and strengthen supply chains in the U.S. and worldwide.
These investments bolster Hitachi Energy’s manufacturing capabilities across the U.S., enhancing production capacity at the company’s transformer factories in Virginia, Missouri, and Mississippi. It includes transformer components such as bushings and insulation as Hitachi Energy is a critical supplier to other transformer manufacturers.
This pivotal move will also help alleviate the broader, ongoing transformer supply shortage, ensuring increased production capacity and supply-chain resilience. The global investment program, one of the most significant in the industry today, also strengthens Hitachi Energy’s manufacturing capabilities in Asia, South America, and Europe.
The demand for transformers and grid infrastructure is rising at an unprecedented scale and pace. As the world’s largest transformer manufacturer, we have a responsibility to expand our capacity and accelerate the delivery of transformers and essential components, helping the industry scale up faster and advance critical infrastructure projects.
Andreas Schierenbeck,
CEO of Hitachi Energy
Transformer investments are part of a broader, multi-billion-dollar investment plan that Hitachi Energy is deploying across its manufacturing, engineering, digital, R&D, and partnership activities. These investments are being rolled out across all major markets globally to enable the company to meet customers’ commitments and market demand. Hitachi Energy is also delivering enhancements in supply chain management, digitalization, and automation, allowing capacity expansion and accelerated speed to market.
Transformers are vital to the clean energy transition and enable the efficient transmission and distribution of electricity. They are a key element in integrating renewables, expanding grid interconnections, powering data centers, electrifying transportation, and facilitating the decarbonization of energy systems.
Transformer insulation and components, such as bushings, tap-changers, insulation material and parts, and other accessories, are essential to the seamless operation of transformers. As the world’s largest transformer manufacturer, Hitachi Energy has over 60 transformer factories and 30 service centers worldwide, providing the world’s broadest portfolio of transformer insulation and components.
Today’s investment builds on the $6 billion USD announced in 2024, including $1.5 billion USD specifically allocated to scaling global transformer production. This expansion is essential to meeting growing demand and supporting long-term decarbonization and electrification efforts.
All announced capacity investments include sustainable and state-of-the-art manufacturing technologies for operational efficiency while ensuring high standards of safety and quality. They are advancing the company’s efforts to become carbon-neutral in its own operations by 2030 and aim to create positive economic and social impacts in local communities wherever the company works.
SourceHitachi Energy
EMR Analysis
More information on Hitachi Energy: See the full profile on EMR Executive Services
More information on Andreas Schierenbeck (Senior Vice President and Executive Officer, Hitachi, Ltd + Chief Executive Officer, Hitachi Energy Ltd): See the full profile on EMR Executive Services
More information on Business Unit Transformers by Hitachi Energy: See the full profile on EMR Executive Services
More information on Bruno Melles (Managing Director, Business Unit Transformers, Hitachi Energy): See the full profile on EMR Executive Services
More information on S&P Global: https://www.spglobal.com/en/ + S&P Dow Jones Indices is the largest global resource for essential index-based concepts, data and research, and home to iconic financial market indicators, such as the S&P 500® and the Dow Jones Industrial Average®. More assets are invested in products based on our indices than products based on indices from any other provider in the world. Since Charles Dow invented the first index in 1884, S&P DJI has been innovating and developing indices across the spectrum of asset classes helping to define the way investors measure and trade the markets.
S&P Dow Jones Indices is a division of S&P Global (NYSE: SPGI), which provides essential intelligence for individuals, companies, and governments to make decisions with confidence.
More information on Douglas L. Peterson (President and Chief Executive Officer, S&P Global): https://www.spglobal.com/en/who-we-are/our-people/operating-committee/douglas-peterson + https://www.linkedin.com/in/douglas-peterson-1254245/
More information on CERAWeek Energy Conference by S&P Global (10th to 14th March 2025 – Houston, USA): https://ceraweek.com/index.html + The CERAWeek community is a unique gathering of top global energy executives and leading members of the industrial, automotive, manufacturing and technology communities, as well as public policy-officials, financial institutions, tech, and thought leaders.
CERAWeek is comprised of three mutually reinforcing platforms: The Executive Conference, the Innovation Agora, and Partner Programs. The industry’s foremost thought leaders convene to cultivate relationships and exchange transformative ideas. Our programs are designed to advance new ideas, insight and solutions to the biggest challenges facing the future of energy, the environment, and climate.
EMR Additional Notes:
- Substation:
- A power station is where the power is generated. A sub station is where power is split apart, distributed and spread further into the grid. A substation is a part of an electrical generation, transmission, and distribution system.
- Substations contain the specialist equipment that allows the voltage of electricity to be transformed (or ‘switched’). The voltage is stepped up or down through pieces of equipment called transformers, which sit within a substation’s site.
- Substations typically include:
- Transformers: The core components for voltage transformation.
- Circuit Breakers: To isolate and protect equipment.
- Switchgear: For controlling and protecting the flow of electricity.
- Shunt Reactors (sometimes): Used to improve system stability.
- Other equipment: Measuring instruments, control panels, etc.
- Transformers (Distribution Transformers, Power Transformers, Traction Transformers and HVDC Converters):
- A distribution transformer is the type of transformer that performs the last voltage transformation in a distribution grid. It converts the voltage used in the transmission lines to one suitable for household and commercial use, typically down to 240 volts.
- The transformer is classified into three types based on the voltage level produced: Step down, Step up, and an isolation transformer.
- Transformers changes from high voltage to low voltage, used in homes and businesses. The main function of this is to reduce the voltage to provide isolation between the two windings as primary and secondary. This transformer distributes electricity to remote areas generated from power plants.
- While transformer stations are linked to high/medium-voltage transmission systems, electrical substations are designed to support and transform lower voltages.
- Distribution transformers always operate at a load less the rated full load. Power transformers always operate at full load. Distribution transformers are designed to give maximum efficiency at 60 to 70% of the rated load. Power transformers have maximum efficiency at full load.
- Power Transformers are used in transmission network of higher voltages for step-up and step down application (400 kV, 200 kV, 110 kV, 66 kV, 33kV) and are generally rated above 200MVA.
- Distribution Transformers are used for lower voltage distribution networks as a means to end user connectivity. (11kV, 6.6 kV, 3.3 kV, 440V, 230V) and are generally rated less than 200 MVA.
- Traction transformers are special transformers used in railway systems to step down high-voltage AC power from the overhead catenary to the required voltage for the train’s traction system. These transformers are typically medium-frequency transformers with ratings ranging from 25 kVA to 25 MVA.
- In HVDC station, the converter transformer steps-up the generated AC voltages to the required level. The converter station takes the electric power from the three-phase AC network and rectifies it to DC, which is then transmitted through overhead lines (or cables).
- Shunt Reactor:
- Shunt reactors (SRs) are used in high voltage energy transmission systems to control the voltage during load variations. Depending on the voltage requirement needs, shunt reactors are switched on or off to provide reactive power compensation.
- A shunt reactor is an absorber of reactive power, thus, increasing the energy efficiency of the system. It is the most compact device commonly used for reactive power compensation in long high-voltage transmission lines and in cable systems. The shunt reactor can be directly connected to the power line or to a tertiary winding of a three-winding transformer. The shunt reactor could be permanently connected or switched via a circuit breaker.
- Shunt reactor is same as power transformer but it has only one winding per phase as compared to power transformer. Shunt reactors are used to increase the power and energy system efficiency as it absorb & compensate the reactive power in cables and long high voltage transmission lines.

- Grid, Microgrids, DERs and DERM’s:
- The power grid is a network for delivering electricity to consumers. The power grid includes generator stations, transmission lines and towers, and individual consumer distribution lines.
- The grid constantly balances the supply and demand for the energy that powers everything from industry to household appliances.
- Electric grids perform three major functions: power generation, transmission, and distribution.
- A microgrid is a small-scale power grid that can operate independently or collaboratively with other small power grids. The practice of using microgrids is known as distributed, dispersed, decentralized, district or embedded energy production.
- Smart Grid is any electrical grid + IT at all levels . Micro Grid is a group of interconnected loads and DERs (Distributed energy resources) within a clearly defined electrical and geographical boundaries witch acts as a single controllable entity with respect to the main grid.
- Distributed energy resources (DERs) are small-scale electricity supply (typically in the range of 3 kW to 50 MW) or demand resources that are interconnected to the electric grid. They are power generation resources and are usually located close to load centers, and can be used individually or in aggregate to provide value to the grid.
- Common examples of DERs include rooftop solar PV units, natural gas turbines, microturbines, wind turbines, biomass generators, fuel cells, tri-generation units, battery storage, electric vehicles (EV) and EV chargers, and demand response applications.
- Distributed energy resources management systems (DERMS) are platforms which helps mostly distribution system operators (DSO) manage their grids that are mainly based on distributed energy resources (DER).
- DERMS are used by utilities and other energy companies to aggregate a large energy load for participation in the demand response market. DERMS can be defined in many ways, depending on the use case and underlying energy asset.
- AI – Artificial Intelligence:
- Artificial intelligence is the simulation of human intelligence processes by machines, especially computer systems.
- As the hype around AI has accelerated, vendors have been scrambling to promote how their products and services use AI. Often what they refer to as AI is simply one component of AI, such as machine learning. AI requires a foundation of specialized hardware and software for writing and training machine learning algorithms. No one programming language is synonymous with AI, but well a few, including Python, R and Java, are popular.
- In general, AI systems work by ingesting large amounts of labeled training data, analyzing the data for correlations and patterns, and using these patterns to make predictions about future states. In this way, a chatbot that is fed examples of text chats can learn to produce lifelike exchanges with people, or an image recognition tool can learn to identify and describe objects in images by reviewing millions of examples.
- AI programming focuses on three cognitive skills: learning, reasoning and self-correction.
- What are the 4 types of artificial intelligence?
- Type 1: Reactive machines. These AI systems have no memory and are task specific. An example is Deep Blue, the IBM chess program that beat Garry Kasparov in the 1990s. Deep Blue can identify pieces on the chessboard and make predictions, but because it has no memory, it cannot use past experiences to inform future ones.
- Type 2: Limited memory. These AI systems have memory, so they can use past experiences to inform future decisions. Some of the decision-making functions in self-driving cars are designed this way.
- Type 3: Theory of mind. Theory of mind is a psychology term. When applied to AI, it means that the system would have the social intelligence to understand emotions. This type of AI will be able to infer human intentions and predict behavior, a necessary skill for AI systems to become integral members of human teams.
- Type 4: Self-awareness. In this category, AI systems have a sense of self, which gives them consciousness. Machines with self-awareness understand their own current state. This type of AI does not yet exist.
- Machine Learning (ML):
- Developed to mimic human intelligence, it lets the machines learn independently by ingesting vast amounts of data, statistics formulas and detecting patterns.
- ML allows software applications to become more accurate at predicting outcomes without being explicitly programmed to do so.
- ML algorithms use historical data as input to predict new output values.
- Recommendation engines are a common use case for ML. Other uses include fraud detection, spam filtering, business process automation (BPA) and predictive maintenance.
- Classical ML is often categorized by how an algorithm learns to become more accurate in its predictions. There are four basic approaches: supervised learning, unsupervised learning, semi-supervised learning and reinforcement learning.
- Deep Learning (DL):
- Subset of machine learning, Deep Learning enabled much smarter results than were originally possible with ML. Face recognition is a good example.
- DL makes use of layers of information processing, each gradually learning more and more complex representations of data. The early layers may learn about colors, the next ones about shapes, the following about combinations of those shapes, and finally actual objects. DL demonstrated a breakthrough in object recognition.
- DL is currently the most sophisticated AI architecture we have developed.
- Computer Vision (CV):
- Computer vision is a field of artificial intelligence that enables computers and systems to derive meaningful information from digital images, videos and other visual inputs — and take actions or make recommendations based on that information.
- The most well-known case of this today is Google’s Translate, which can take an image of anything — from menus to signboards — and convert it into text that the program then translates into the user’s native language.
- Machine Vision (MV):
- Machine Vision is the ability of a computer to see; it employs one or more video cameras, analog-to-digital conversion and digital signal processing. The resulting data goes to a computer or robot controller. Machine Vision is similar in complexity to Voice Recognition.
- MV uses the latest AI technologies to give industrial equipment the ability to see and analyze tasks in smart manufacturing, quality control, and worker safety.
- Computer Vision systems can gain valuable information from images, videos, and other visuals, whereas Machine Vision systems rely on the image captured by the system’s camera. Another difference is that Computer Vision systems are commonly used to extract and use as much data as possible about an object.
- Generative AI (GenAI):
- Generative AI technology generates outputs based on some kind of input – often a prompt supplied by a person. Some GenAI tools work in one medium, such as turning text inputs into text outputs, for example. With the public release of ChatGPT in late November 2022, the world at large was introduced to an AI app capable of creating text that sounded more authentic and less artificial than any previous generation of computer-crafted text.
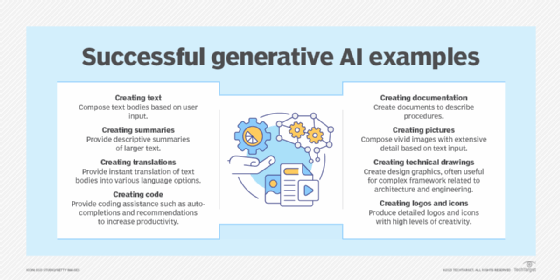
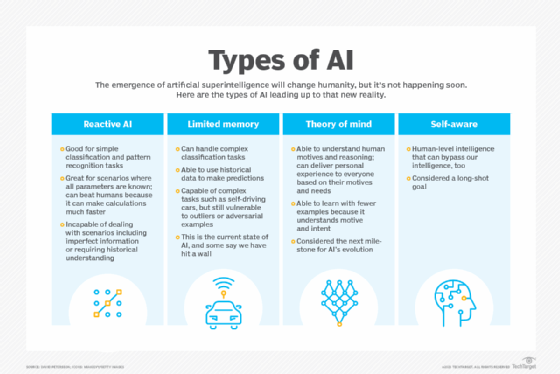
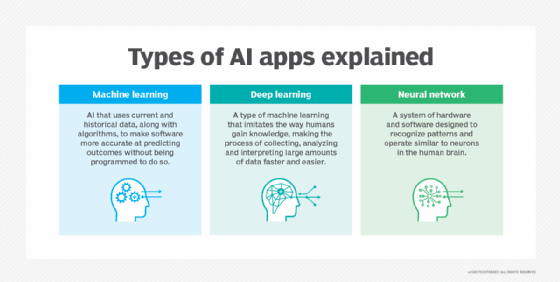
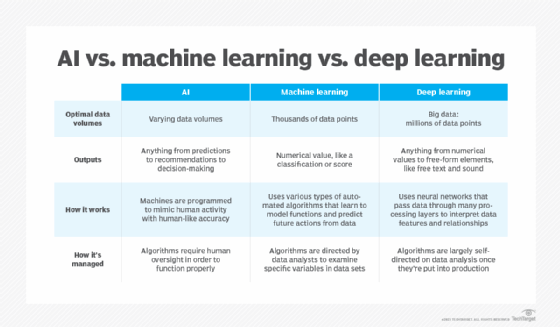
- Edge AI Technology:
- Edge artificial intelligence refers to the deployment of AI algorithms and AI models directly on local edge devices such as sensors or Internet of Things (IoT) devices, which enables real-time data processing and analysis without constant reliance on cloud infrastructure.
- Simply stated, edge AI, or “AI on the edge“, refers to the combination of edge computing and artificial intelligence to execute machine learning tasks directly on interconnected edge devices. Edge computing allows for data to be stored close to the device location, and AI algorithms enable the data to be processed right on the network edge, with or without an internet connection. This facilitates the processing of data within milliseconds, providing real-time feedback.
- Self-driving cars, wearable devices, security cameras, and smart home appliances are among the technologies that leverage edge AI capabilities to promptly deliver users with real-time information when it is most essential.
- Multimodal Intelligence and Agents:
- Subset of artificial intelligence that integrates information from various modalities, such as text, images, audio, and video, to build more accurate and comprehensive AI models.
- Multimodal capabilities allows to interact with users in a more natural and intuitive way. It can see, hear and speak, which means that users can provide input and receive responses in a variety of ways.
- An AI agent is a computational entity designed to act independently. It performs specific tasks autonomously by making decisions based on its environment, inputs, and a predefined goal. What separates an AI agent from an AI model is the ability to act. There are many different kinds of agents such as reactive agents and proactive agents. Agents can also act in fixed and dynamic environments. Additionally, more sophisticated applications of agents involve utilizing agents to handle data in various formats, known as multimodal agents and deploying multiple agents to tackle complex problems.
- Small Language Models (SLM) and Large Language Models (LLM):
- Small language models (SLMs) are artificial intelligence (AI) models capable of processing, understanding and generating natural language content. As their name implies, SLMs are smaller in scale and scope than large language models (LLMs).
- LLM means large language model—a type of machine learning/deep learning model that can perform a variety of natural language processing (NLP) and analysis tasks, including translating, classifying, and generating text; answering questions in a conversational manner; and identifying data patterns.
- For example, virtual assistants like Siri, Alexa, or Google Assistant use LLMs to process natural language queries and provide useful information or execute tasks such as setting reminders or controlling smart home devices.
- Bushing Style:
- Bushings are cylindrical bearings that are used to reduce friction between moving parts so “bushing style” refers to a design feature related to internal bearings within the pump.
- Carbon Dioxide (CO2):
- Primary greenhouse gas emitted through human activities. Carbon dioxide enters the atmosphere through burning fossil fuels (coal, natural gas, and oil), solid waste, trees and other biological materials, and also as a result of certain chemical reactions (e.g., manufacture of cement). Carbon dioxide is removed from the atmosphere (or “sequestered”) when it is absorbed by plants as part of the biological carbon cycle.
- Biogenic Carbon Dioxide (CO2):
- Biogenic Carbon Dioxide (CO2) and Carbon Dioxide (CO2) are the same. Scientists differentiate between biogenic carbon (that which is absorbed, stored and emitted by organic matter like soil, trees, plants and grasses) and non-biogenic carbon (that found in all other sources, most notably in fossil fuels like oil, coal and gas).
- Decarbonization:
- Reduction of carbon dioxide emissions through the use of low carbon power sources, achieving a lower output of greenhouse gasses into the atmosphere.
- Carbon Footprint:
- There is no universally agreed definition of what a carbon footprint is.
- A carbon footprint is generally understood to be the total amount of greenhouse gas (GHG) emissions that are directly or indirectly caused by an individual, organization, product, or service. These emissions are typically measured in tonnes of carbon dioxide equivalent (CO2e).
- In 2009, the Greenhouse Gas Protocol (GHG Protocol) published a standard for calculating and reporting corporate carbon footprints. This standard is widely accepted by businesses and other organizations around the world. The GHG Protocol defines a carbon footprint as “the total set of greenhouse gas emissions caused by an organization, directly and indirectly, through its own operations and the value chain.”
- CO2e (Carbon Dioxide Equivalent):
- CO2e means “carbon dioxide equivalent”. In layman’s terms, CO2e is a measurement of the total greenhouse gases emitted, expressed in terms of the equivalent measurement of carbon dioxide. On the other hand, CO2 only measures carbon emissions and does not account for any other greenhouse gases.
- A carbon dioxide equivalent or CO2 equivalent, abbreviated as CO2-eq is a metric measure used to compare the emissions from various greenhouse gases on the basis of their global-warming potential (GWP), by converting amounts of other gases to the equivalent amount of carbon dioxide with the same global warming potential.
- Carbon dioxide equivalents are commonly expressed as million metric tonnes of carbon dioxide equivalents, abbreviated as MMTCDE.
- The carbon dioxide equivalent for a gas is derived by multiplying the tonnes of the gas by the associated GWP: MMTCDE = (million metric tonnes of a gas) * (GWP of the gas).
- For example, the GWP for methane is 25 and for nitrous oxide 298. This means that emissions of 1 million metric tonnes of methane and nitrous oxide respectively is equivalent to emissions of 25 and 298 million metric tonnes of carbon dioxide.
- Carbon Capture and Storage (CCS) – Carbon Capture, Utilisation and Storage (CCUS):
- CCS involves the capture of carbon dioxide (CO2) emissions from industrial processes. This carbon is then transported from where it was produced, via ship or in a pipeline, and stored deep underground in geological formations.
- CCS projects typically target 90 percent efficiency, meaning that 90 percent of the carbon dioxide from the power plant will be captured and stored.
- Carbon Dioxide Removal (CDR):
- Carbon Dioxide Removal encompasses approaches and methods for removing CO2 from the atmosphere and then storing it permanently in underground geological formations, in biomass, oceanic reservoirs or long-lived products in order to achieve negative emissions.
- Direct Air Capture (DAC):
- Technologies extracting CO2 directly from the atmosphere at any location, unlike carbon capture which is generally carried out at the point of emissions, such as a steel plant.
- Constraints like costs and energy requirements as well as the potential for pollution make DAC a less desirable option for CO2 reduction. Its larger land footprint when compared to other mitigation strategies like carbon capture and storage systems (CCS) also put it at a disadvantage.
- Carbon Credits or Carbon Offsets:
- Permits that allow the owner to emit a certain amount of carbon dioxide or other greenhouse gases. One credit permits the emission of one ton of carbon dioxide or the equivalent in other greenhouse gases.
- The carbon credit is half of a so-called cap-and-trade program. Companies that pollute are awarded credits that allow them to continue to pollute up to a certain limit, which is reduced periodically. Meanwhile, the company may sell any unneeded credits to another company that needs them. Private companies are thus doubly incentivized to reduce greenhouse emissions. First, they must spend money on extra credits if their emissions exceed the cap. Second, they can make money by reducing their emissions and selling their excess allowances.